Tungsten Sulfide
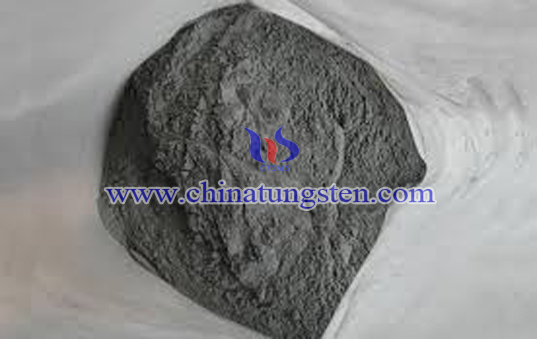
Tungsten(IV) sulfide is the chemical compound with the formula WS2. It occurs naturally as the rare mineral called tungstenite. This material is a component of certain catalysts used for hydrodesulfurization and hydrodenitrification.
WS2 adopts a layered structure related to MoS2, with W atoms situated in trigonal prismatic coordination sphere. Owing to this layered structure, WS2 forms inorganic nanotubes, which were discovered on an example of WS2 in 1992.
Properties
Bulk WS2 forms dark gray hexagonal crystals with a layered structure. Like the closely related MoS2, it exhibits properties of a dry lubricant. It is chemically fairly inert but is attacked by a mixture of nitric and hydrofluoric acids. When heated in oxygen-containing atmosphere, WS2 converts to tungsten trioxide. When heated in absence of oxygen, WS2 does not melt but decomposes to tungsten and sulfur, but only at 1250 °C.
The material undergoes exfoliation by treatment with various reagents such as chlorosulfonic acid.
Synthesis
WS2 is produced by a number of methods. Many of these methods involve treating oxides with sources of sulfide or hydrosulfide, supplied as hydrogen sulfide or generated in situ. Other routes entail thermolysis of tungsten(VI) sulfides (e.g., (R4N)2WS4) or the equivalent (e.g., WS3).
Applications
WS2 is used, in conjunction with other materials, as catalyst for hydrotreating of crude oil.
Research
Like MoS2, Nanostructured WS2 is heavily studied for potential applications. It has been discussed for the storage of hydrogen and lithium. As such it is of interest to research on material for solid-state secondary lithium battery cathodes and other electrochemical devices. WS2 also catalyses hydrogenation of carbon dioxide:
CO2 + H2 → CO + H2O
Nanotubes
Illustration of PbI2/WS2 core–shell nanostructure.
Tungsten disulfide is the first material which was found to form inorganic nanotubes, in 1992. This ability is related to the layered structure of WS2, and macroscopic amounts of WS2 have been produced by the methods mentioned above. WS2 nanotubes have been investigated as reinforcing agents to improve the mechanical properties of polymeric nanocomposites. In a study, WS2 nanotubes reinforced biodegradable polymeric nanocomposites of polypropylene fumarate (PPF) showed significant increases in the Young's modulus, compression yield strength, flexural modulus and flexural yield strength, compared to single- and multi-walled carbon nanotubes reinforced PPF nanocomposites, suggesting that WS2 nanotubes may be better reinforcing agents than carbon nanotubes. The addition of WS2 nanotubes to epoxy resin improved adhesion, fracture toughness and strain energy release rate. The wear of the nanotubes-reinforced epoxy is lower than that of pure epoxy. WS2 nanotubes were embedded into a poly(methyl methacrylate) (PMMA) nanofiber matrix via electrospinning. The nanotubes were well dispersed and aligned along fiber axis. The enhanced stiffness and toughness of PMMA fiber meshes by means of inorganic nanotubes addition may have potential uses as impact-absorbing materials, e.g. for ballistic vests.
WS2 nanotubes are hollow and can be filled with another material, to preserve or guide it to a desired location, or to generate new properties in the filler material which is confined within a nanometer-scale diameter. To this goal, inorganic nanotube hybrids were made by filling WS2 nanotubes with molten lead, antimony or bithmuth iodide salt by a capillary wetting process, resulting in PbI2@WS2, SbI3@WS2 or BiI3@WS2 core–shell nanotubes.
Nanosheets
WS2 can also exist in the form of atomically thin sheets. Such materials exhibit room-temperature photoluminescence in the monolayer limit